Deflection units in solid-state battery manufacturing
Solid-state batteries have the potential to revolutionise the battery and electric vehicle industry
Solid-state batteries have the potential to revolutionise the battery and electric vehicle industry
Driven by advances in electromobility, large investments have been made in developing this technology over recent years. Today, it is conceivable that the solid-state battery could complement or replace the conventional lithium-ion battery in various applications. The key benefits of this new technology stem from the potentially much higher energy density of the batteries, coupled with their improved safety features and a longer lifetime thanks to reduced cyclic ageing.
However, the development of solid-state batteries is still in its infancy. Pioneering companies in this field include QuantumS- cape in California, which is backed by Volkswagen, and Solid Power, which counts BMW and Ford among its investors. Across the globe, a large number of start-ups, universities and institutes are also researching the future development of solid-state technology – in many cases, supported by the automotive industry. One particular challenge is how to convert the results achieved in the laboratory into commercially feasible cell sizes. Another is how to develop suitable manufacturing techniques. Progress is already been made on both fronts. Just recently, QuantumScape presented a multi-layer solid-state cell format that comes closer to industrially relevant cell formats. “What seemed for a long time to be nothing more than a theoretical technology concept could soon become a reality”, says Dr. Jan Bernd Habedank, Head of the Technical Competence Center at RAYLASE.
According to Johannes Kriegler, research associate at the Institute for Machine Tools and Industrial Management (iwb) of the Technical University of Munich (TUM): “It is now more important than ever to integrate material sciences and manufacturing processes”. Kriegler is currently working on the ProFeLi project, funded by the Federal Ministry of Education and Research (BMBF), to design manufacturing techniques for solid-state batteries with a lithium metal anode. He is specifically investigating the scalability of manufacturing processes for solid-state cells, with a particular focus on the use of modern laser processes. Quality and cost-efficiency are essential. A partially automated research and production plant with an integrated laser system and RAYLASE deflection unit for laser cutting of the innovative battery components was recently developed and put into operation
The goal is clear – the researchers want to leverage the bene- fits of the highly flexible and contact-free scanner technology to move the manufacturing of solid-state batteries a step closer to series production.
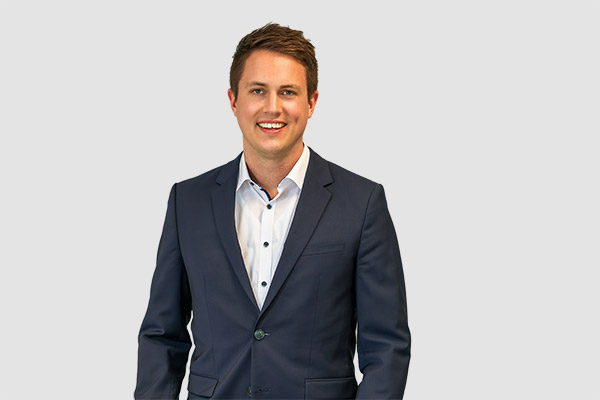
Dr. Jan Bernd Habedank, Head of Technical Competence Center
HOW DOES A SOLID-STATE BATTERY DIFFER FROM A LITHIUM-ION BATTERY?
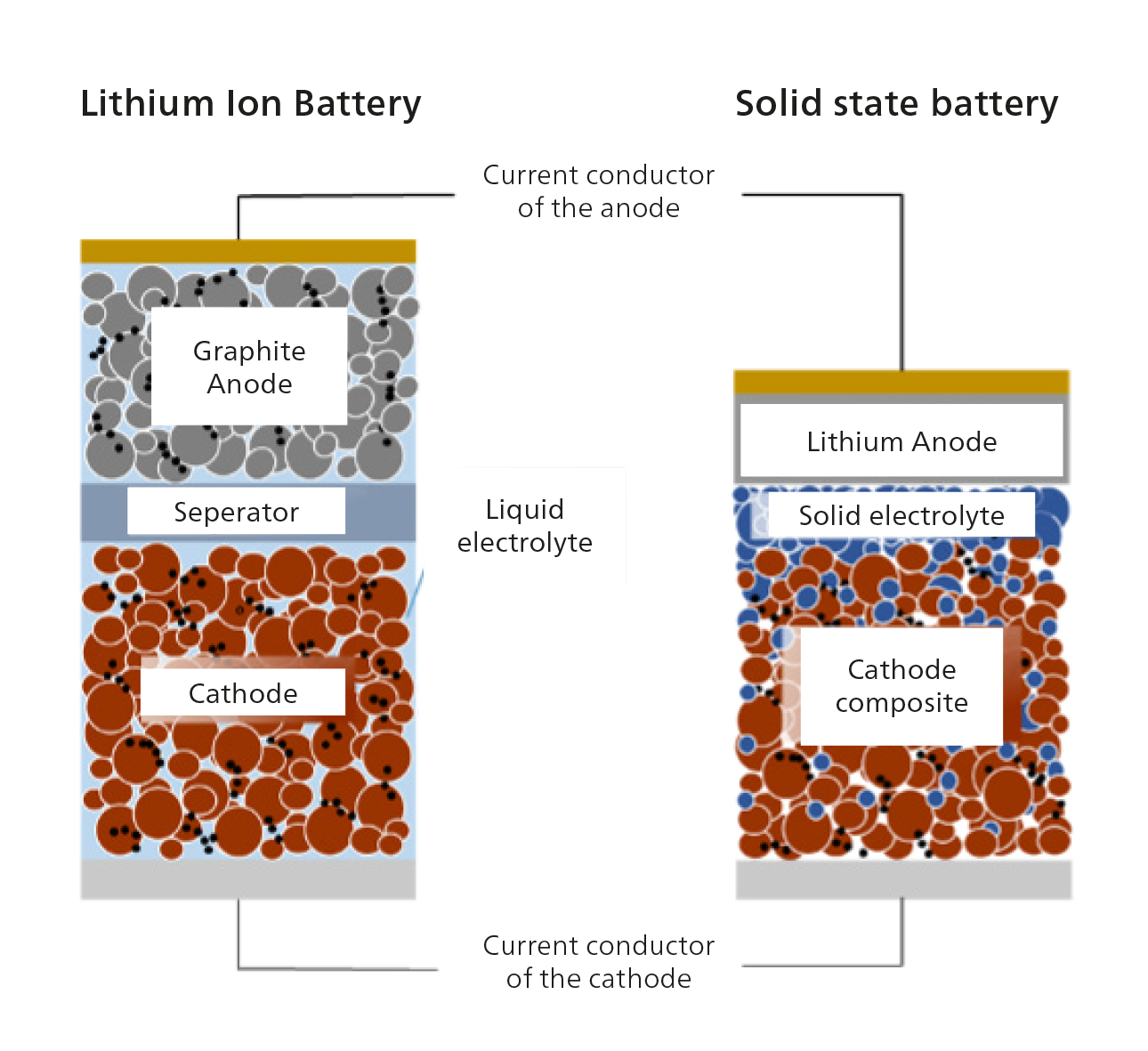
Schematic structure of a lithium ion battery and a solid-state battery
In order to understand the particular benefits and chal- lenges of solid-state batteries, it is useful to compare them with conventional lithium-ion batteries. In both cases, the smallest unit (or elementary cell) consists of two electrodes. In conventional cells, a copper foil coated with graphite serves as an anode, while an aluminium foil coated with a lithium metal oxide serves as a cathode. The electrodes are electrically separated by a microporous separator. A liquid, ion-conducting electrolyte permeates the porous electrodes and the separator, and enables the exchange of lithium ions within the cell. Meanwhile, the cell conductors allow the electrical current generated to be tapped. In contrast, lithium ion transport in a solid-state battery is enabled by a solid electrolyte. The solid electrolyte is also located in the cathode composite (see graphic above) to ensure ion conductivity there too.
The solid electrolyte simultaneously acts as a mechanical barrier between the anode and cathode. This means that metallic lithium can be used as an anode instead of a graphite-coated copper foil. This type of lithium anode can be much slimmer, which saves space in the cell and ultimately increases energy density per cell volume. Without the solid separation layer, this anode design would not be possible for safety reasons.
But how can these new materials be processed during manufacturing? And how can the electrodes and separators be cut to the required sizes with the correct contours? This is where laser systems and intelligent deflection units come into play, as these are essential to enabling high-precision, flexible processes at high speed – all of which will be crucial in future series production.
Product solutions:
RAYLASE offers optimised laser beam deflection units with a wide range of configuration options for contour cutting oflayer components for solid-state batteries. The RAYLASE SUPERSCAN-IV-15 or AXIALSCAN-II-50 is ideal, depending on the required field size.
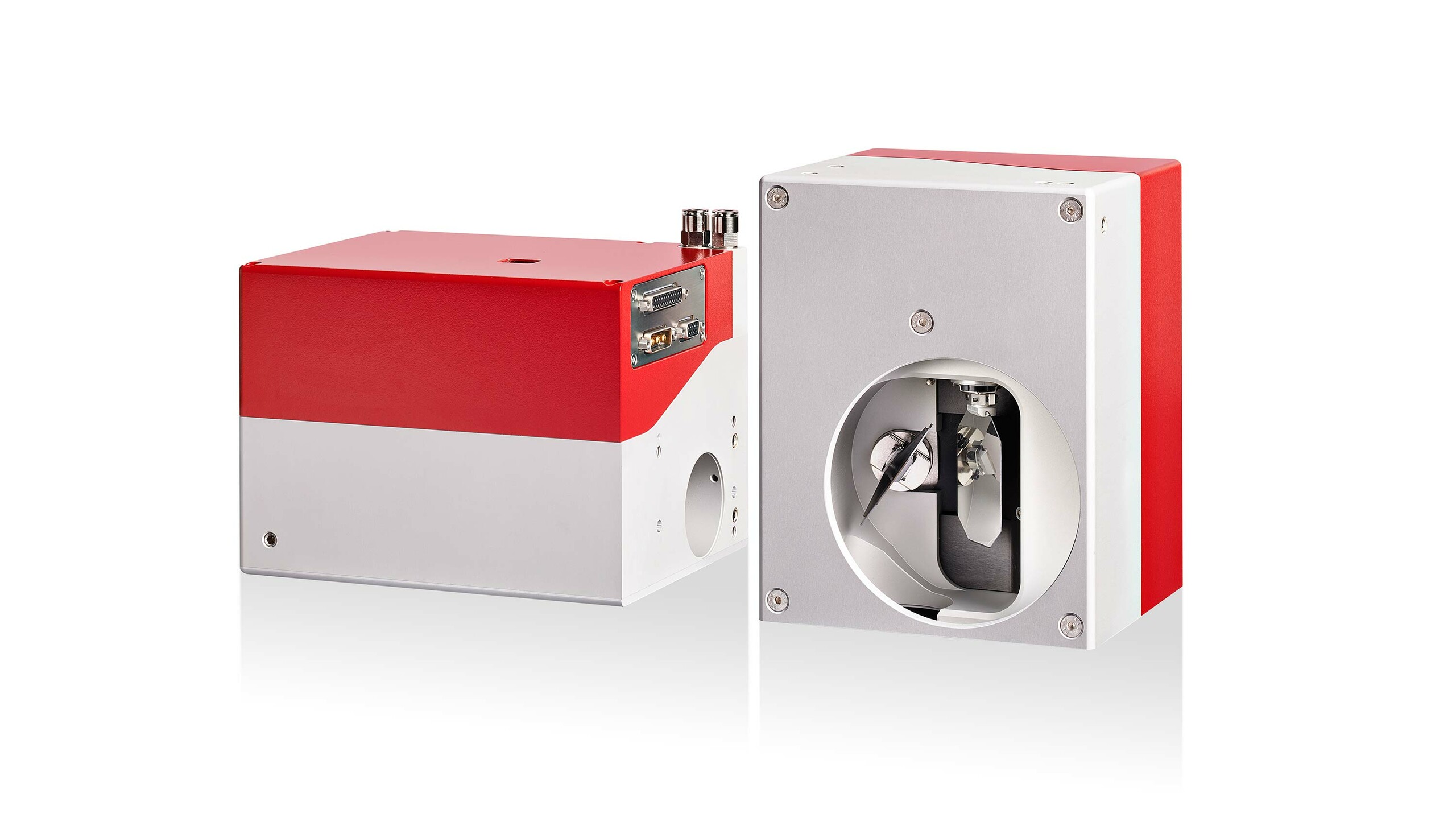
Degradation due to contact with moisture
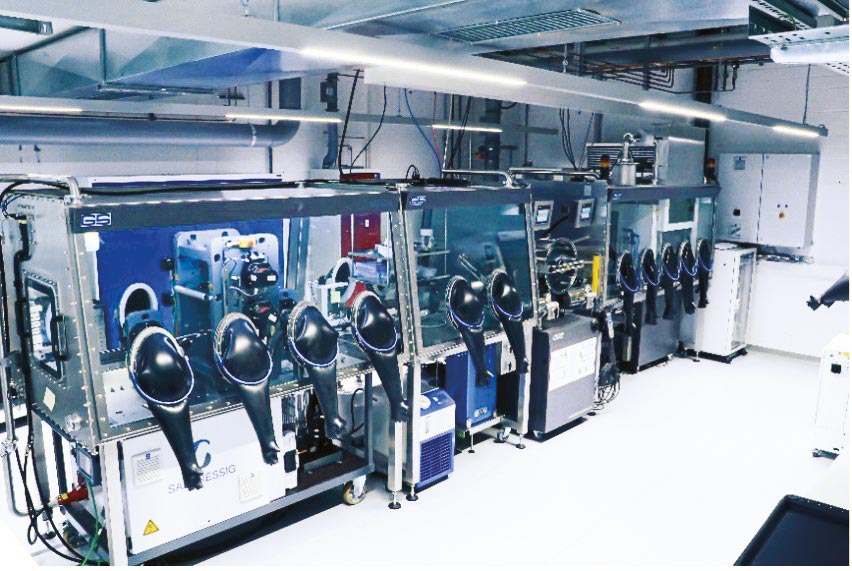
Quelle: iwb/TUM
Johannes Kriegler: “In research, the usual procedure is to begin by ensuring that the material is set out flawlessly, before developing a suitable product design and finally defining the steps involved in production. In this case, however, we need to take a holistic approach because each of these elements is dependent on the others. For example, there are many challenges to overcome when processing the foil-like lithium metal layer. The material is extremely difficult to handle, as it has a soft consistency and tends to be very sticky. Under no circumstances can the lithium be allowed to come into contact with moisture or water during any of the individual manufacturing steps. Otherwise, a strong oxidation reaction occurs because the lithium degrades and, as a result, can no longer be used in a battery. For this reason, production usually takes place under shielding gas or in a dry room.
Solutions: IWB PILOT LINE FOR THE PRODUCTION OF SOLID-STATE BATTERIES
The iwb at TUM has set up a laboratory line to assemble solid-state batteries under special conditions. The actual production of solid-state cells occurs in an argon atmosphere within an enclosure known as a glove box, which can be flooded with shielding gas. The benefit is that gas prevents possible reactions in the materials, which would otherwise normally occur as a result of contact with moisture and nitrogen in the air. While the laser beam source is located outside of the glove box, the RAYLASE SUPERSCAN-IV-15 deflection unit is integrated into the box’s interior. The laser beam is guided into the glove box through a laser protective glass window. Johannes Kriegler: “RAYLASE impressed us – not only with their product but also with their service and with how easy their technology is to use in this difficult environment. The on-axis camera module was a real advantage, as this makes it easy to set up the process with the “Click&Teach” function and control it with the software”.
The laser beam is guided into the argon glove box and into the deflection unit via a mirror system and a laser protective window. Image source iwb.
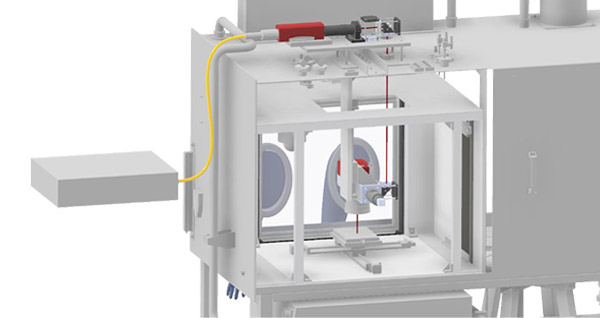
Quelle: iwb
When setting up the pilot line, a large degree of flexibility was built in so that various types of material can be studied. Thanks to the flexible structure of the system, the researchers can put specific electrolyte materials to the test – including glasses, ceramics, polymers, and multi-material systems. At present, research is being conducted on all material “fronts” across the globe, as it is not yet clear which materials will prevail in future. The material of choice may depend on the focus and the application. For example, a battery cell for use in a leaf blower may require a different material to one intended to be used in an artificial cardiac pacemaker or an electric vehicle. The electrolyte material used will also affect the potential gain in terms of safety that the new technology will bring. As Kriegler points out “Solid-state cells will be saf- er and more reliable, as they don’t contain flammable liquid electrolyte”. After all, one of the challenges associated with conventional lithiumion batteries is the growth of lithium dendrites. These are small, pointed lithium metal deposits that can pierce through the battery separator, causing a short circuit in the battery cell. In solid-state batteries, this effect can be suppressed by means of the mechanical electrolyte barrier.
RAYLASE-CEO Dr. Philipp Schön: “It’s important for us to be involved even at this early stage of solid-state battery development. We’re confident that high-speed cutting of solid-state cell components will become indispensable to production, as competing techniques reach their limits. The speed and precision of pulsed lasers with a deflection unit like the AXIALSCAN-II-50 or SUPERSCAN-IV-15 are unrivalled”.
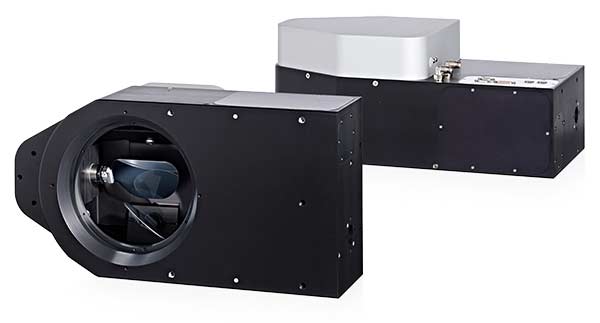
RAYLASE solution - AXIALSCAN II-50
With its mirrors for a 50 mm beam size, the AXIALSCAN-II-50 can generate an exceptionally small laser spot diameter, even in large processing fields. This produces a very clean and even cutting edge in electrodes and electrolyte layers with a small heat affected zone. In addition, the extremely light yet stiff deflection mirrors enable highly dynamic responses, so that even 90° changes in direction during cutting of the solid electrolyte layers and electrodes can be executed precisely and without burr formation.
The deflection unit is also supported by SP-ICE-3 control electronics and the new RAYGUIDE software, both from RAYLASE. These enable a range of functions with real-time capability, specially designed to meet the requirements of battery cell production.
For example, RAYGUIDE laser software offers an optimised customer interface with the control electronics and, if required, an intuitive yet highly functional process configuration interface. It clearly visualises the processes and indicates when they are executed with a position-accurate time-stamp. In addition, the control electronics are also capable of exact consideration of line speeds (detected by encoders) – even during speed deceleration – and of performing cuts in precise positions. This function is known as MOTF – marking on the fly.
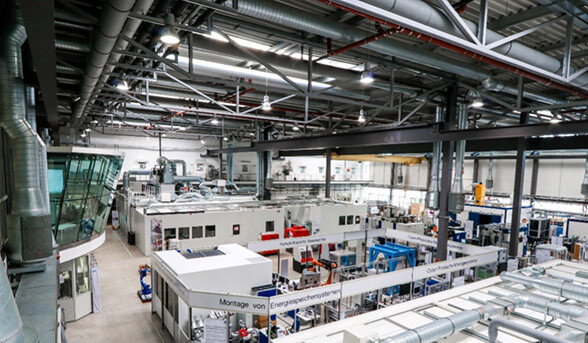
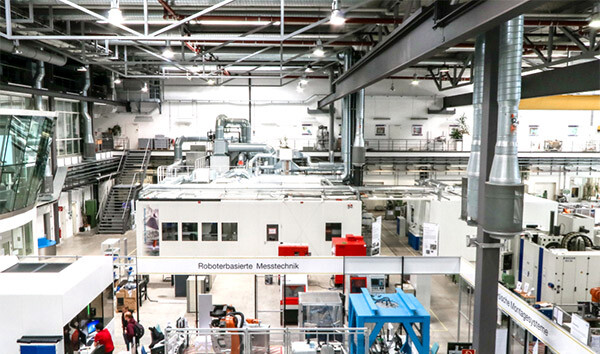
iwb testing hall (source: iwb/TUM)
iwb TUM perspectives for future production
The Institute for Machine Tools and Industrial Manage- ment (iwb) of the Technical University of Munich is one of Germany’s largest research institutes for manufacturing technology. It encompasses three chairs at the TUM School of Engineering and Design in Garching near Munich – the Chair for Industrial Management and Assembly Technologies, the Chair for Machine Tools and Manufacturing Technology and the Chair for Production Technology and Energy Storage Systems. The Battery Production department is involved in researching the manufacturing of innovative lithium-ion and solid-state battery cells. The focus of the research is process development and optimisation of the process chain within battery production. From mixing the electrode materials to the first charging and discharging cycles of the manufactured cells and battery module assembly, all steps are carried out in-house on the iwb research production line. For more than five years, the department has been researching a wide range of materials used in conventional lithium-ion batteries, as well as the use of metallic lithium in solid-state batteries. Johannes Kriegler says “My vision is to enable cost-efficient production of solid-state batteries by using innovative manufacturing processes and plant engineering, thereby contributing to a greener future”.
After all, the yardstick for successful solid-state technology – and all technology – must be a competitive and cost-efficient manufacturing process.